I was diagnosed with coronavirus in September 2020. Here is what I discovered.
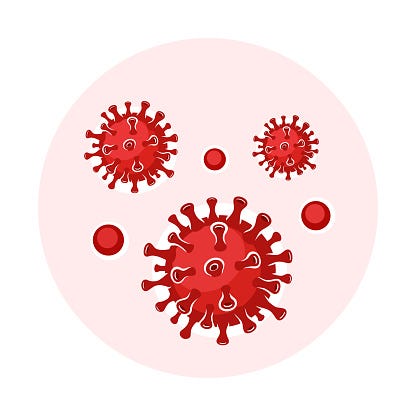
The immediate effects of coronavirus were expected. Fatigue, loss of taste, muscle aches, headaches but no chest pain or persistent cough. As such my symptom list was shorter and less intense than most people have suffered suggesting that symptoms vary according to vulnerability.
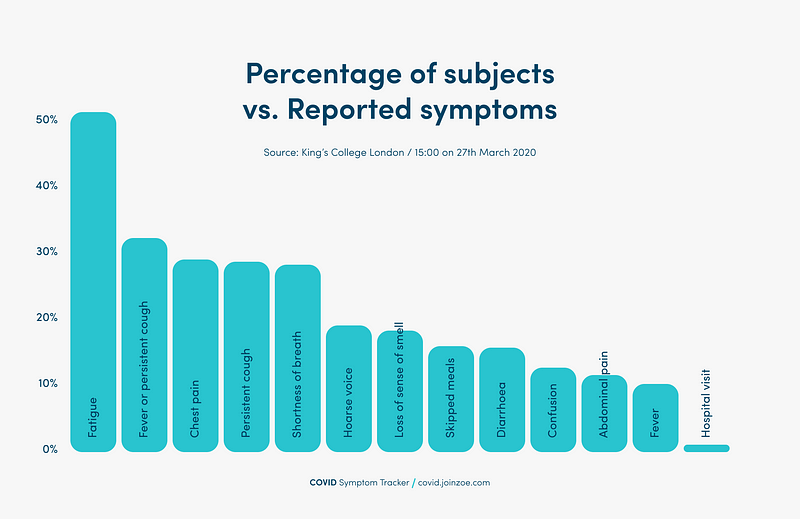
Less anticipated were the effects on cardiovascular performance, ie a combination of deconditioning and detraining. I saw a dramatic reduction of power, accompanied by comparable reduction in motivation. This aligns with studies which have shown a 10% loss in VO2 max after 10 days and a 25% loss of VO2 max after 25 days of bed rest.
If unwell with a serious illness such as coronavirus, rest seems sensible for sure, indeed social exercise might be restricted, but how long should you rest for? Isolation usually lasts 10 days but there is a temptation to rest for much longer especially when you feel your previous bests are out of reach. In the past, unwell hospitalized patients were told to rest and often spent > 80% of their hospital admission confined to their bed but increasingly this extended inactivity became recognized as potentially hazardous for recovery.
Let’s briefly examine the process of deconditioning and ask what, if anything can be done to minimize these deleterious effects?
Deconditioning and Plasma Volume
Even after a short period of rest, the cardiovascular system undergoes extensive changes. Rest and particularly bedrest for longer than 24 hours results in a shift of around 1L fluid from the legs to the chest which increases intracardiac pressure. This stimulates the release of atrial natriuretic peptide (ANP). This initiates diuresis, leading to significant water loss and sodium excretion by the kidneys that further contributes to the reduction in plasma volume.
Deconditioning and Heart Rate
According to the Frank-Starling principle, the greater the volume of blood entering the heart, the greater the volume ejected. With progressive reductions in plasma/blood volume there is a gradual reduction in the diastolic volume and stroke volume. To maintain sufficient cardiac output, heart rate increases. After four weeks of bedrest, the resting heart rate typically increases by around 10bpm. Similarly, heart rate after equivalent exercise is up to 40bpm faster in patients who have just had a period of bedrest because oxygen carrying capacity is low.
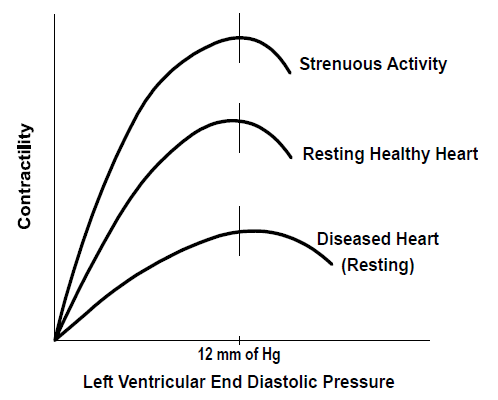
Magnetic resonance imaging and echocardiography has shown that after 4–6 weeks of bedrest, the total mass of the left ventricle of the heart decreases by around 8% and after 8 weeks there is an equivalent change in the right ventricle (Perhonen et al, 2001, Pedlar et al, 2018).
Deconditioning and Skeletal muscles
The skeletal muscles of the legs, particularly the calf muscles, play an important role in compressing the major veins in the legs. This helps to force blood upwards against the natural pull of gravity, ensuring adequate venous return to the heart. Prolonged bedrest rapidly leads to skeletal muscle atrophy (sarcopenia) throughout the body . After bedrest, calf and quadriceps muscles showed a volume reduction of 16% after 20 days and 45 % after 90 days. Muscle fibres become less sensitive to insulin and, as a result, there is less glucose to power muscular contraction.
Deconditioning and V02 Max /Fitness
Following reductions in blood/plasma volume and stroke volume, heart rate reserve is impaired resulting in reduced fitness. At least 80 studies have been published suggesting bed rest impairs V̇o2max. In this research, the absolute V̇o2max (l/min) declined at 0.3% per day per day during bed rest (LINK)
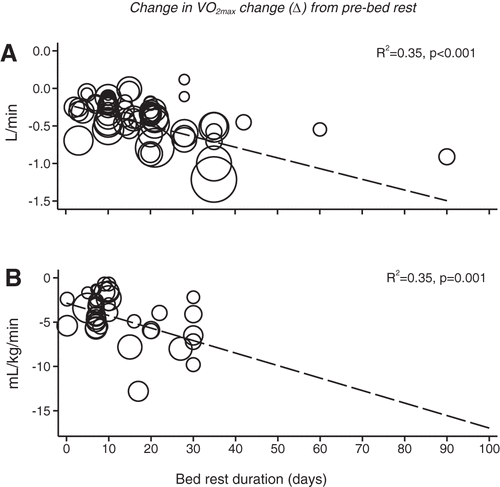
Mechanisms are thought to include reduced plasma volume, reduced red blood cell mass, blunted vasodilator function, cardiac atrophy, and decreased peripheral O2 diffusing capacity. With time there are changes in capillary density and reductions in mitochondria. Muscle and blood glycogen levels (carbohydrate storage) decrease .
Collectively these changes result in a reduced tolerance of exercise that is typically low for months after rehabilitation begins (Corcoran, 1991).
Countermeasures to Prevent Deterioration
A study from the Hong Kong flu epidemic of 1997 demonstrated that patients who regularly performed ‘low/moderate exercise’ had a significantly lower risk of mortality than patients who ‘never/seldom’ exercised (odds ratio 0.62) (link)
During rehabilitation, physical activity is probably the most effective countermeasure either in the form of aerobic or anaerobic exercise (Holt et al., 2016; Demontis et al., 2017). Fifty-eight studies trialled preventive use of a spin bike/ergometer. Recent findings also show high-intensity interval training (HIIT) improves aerobic capacity, endothelial, and left-ventricular function, vasomotor function, and blood pressure (Hussain et al., 2016) following illness. For example daily rowing ergometry and biweekly strength exercise training after 5 weeks of HDT prevented orthostatic intolerance only when combined with volume loading (Hastings et al., 2012).
Summary
Coronavirus is a serious condition and isolation is strongly recommended. Social distancing alone has led to a 9% reduction in weekly activity levels across the UK (link). The illness itself is much more pervasive. However, complete rest is usually not the best option. Remaining as active as possible reduces deterioration and is a protective factor for mortality in general